Introduction
This lecture presents a review of anaerobic metabolism.
Glycolysis - overview
Glucose is metabolised in the cell through glycolysis, also know as
the Embden-Meyerhoff Pathway
"Glycolysis is the primary pathway for anaerobic degradation of
D-glucopyranoses and other D-hexopyranoses. It is probably universal among
organisms: certainly the enzymes which catalyze the pathway's reactions
are among the most conserved (and therefore presumably most ancient) among
proteins." (Quoted from ref. 3 below).
Under anaerobic conditions, glycolysis is a self-contained process leading to the production of fermentation products which vary from organism to organism. In mammalian cells, the primary product is lactate; in yeasts, ethanol and CO2. The process can be split into several stages:
- "Activation" of glucose
- Glucose is first phosphorylated to form glucose-6-Pi, then isomerised
into fructose-6-Pi. The phosphorylation reactions requires ATP. This series of reactions serves two main purposes:
- the glucose is "activated" so as to be able to enter the
pathway;
- the glucose is removed from solution in the cytoplasm, thus lowering
the concentration and favoring the transport gradient into the cell.
- Formation of triose phosphates
- The fructose-6-Pi is phosphorylated again to give fructose-1,6-bisphosphate,
using another ATP, and then split into two triose-Pi molecules, dihydroxyacetone
phosphate (DHAP) and glyceraldehyde-3-Pi, using the enzyme aldolase. The
triose phosphates are interconvertible through triose phosphate isomerase.
- Substrate level phosphorylation through oxidation of glyceraldehyde-3-phosphate
- Glyceraldehyde-3-Pi is oxidized in a reaction in which phosphate is
bound, and NAD+ is reduced to NADH. The free-energy of the oxidation
reaction is used to form a phosphate bond with a high negative free energy
of hydrolysis (a "high-energy" bond). The 1,3-bisphosphoglycerate
is then converted to 3-phosphoglycerate by a kinase reaction in which the
"high-energy" phosphate on the carboxylate end is transfered
to ADP to form ATP.
- ATP synthesis linked to conversion of phosphoenolpyruvate to pyruvate
- 3-phosphoglycerate is converted to 2-phosphoglycerate by phosphoglycerate
mutase, and then dehydrated to give phosphoenolpyruvate, using the enzyme
enolase. This converts the phosphate bond at the 2-position to a "high-energy"
bond.
- The phosphoenolpyruvate reacts with ADP to form ATP and pyruvate, using
pyruvate kinase
- Reduction of pyruvate to regenerate NAD+
- In order to restore the NAD+ used up in glyceraldehyde-3-Pi
oxidation, the pyruvate is reduced to lactate using NADH
The net yield of anaerobic glycolysis is 2ATP / glucose, with an overall
reaction:
glucose + 2 ADP + 2 phosphate <==> 2 lactate + 2 ATP
Central Role of ATP in energy metabolism
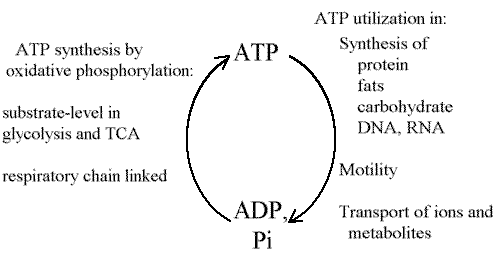
The conversion between ATP, and ADP and phosphate, plays a central role
in the energy metabolism of the cell. The poise of the reaction in a metabolic
compartment plays a determining role in the direction of metabolism, either
directly through thermodynamics, or indirectly through the activating (or
inhibitory) effects on enzymes.
Compartmentalization of the eukaryote cell
- Cytoplasmic metabolism probably reflects an archeal origin
- Mitochondrial structure and eubacterial origin
- Distribution of metabolic activities between cytoplasmic and mitochondrial
compartments
Useful Links
Glycolysis
A nice
introduction to glycolysis
- A
clickable pathway with biochemical information, PDB files of enzymes*
- Glycolysis in a Chime tutorial.
- A
clickable metabolic web representation of glycolysis, which provides links
to reaction parameters, physical chemistry, PDB files of intermediates*,
etc.(The Klotho project)
*(You will need a viewer to see Brookhaven Protein Data
Bank (PDB) structural files. If you use Netscape, you can download Rasmol
(a stand-alone viewer) or Chime (a plug-in) by clicking here. Download
Rasmol, or Chime
plug-in)
- What Is There? (WIT- a successor to the PUMA project).
- A
text version with clickable reactions
- A
colorful summary of glycolysis and the citric acid cycle
©Copyright 1996,
Antony Crofts, University of Illinois at Urbana-Champaign,
a-crofts@uiuc.edu