Lecture 24Secondary electron transport in photosynthesis
|
Secondary acceptors
Bacterial reaction centers - the backreaction
Measurement of the equilibrium constants in the two-electron gate from the rates of the back reactions.
Photosystem II - The two-electron gates for reduction of quinone
The two-electron gate of photosystem II and the mechanism of herbicide resistance
Structure of Photosystem II
See Lecture 22 for a tutorial on the structure of photosystem II.
The FeS centers of photosystem I
See Lecture 22 for a tutorial on the structure of photosystem I.
Donor reactions
Cytochrome c2 as donor to bacterial reaction centers
Cytochrome (cyt) c2 is the donor to (BChl)2+ in phototrophic purple bacteria which do not have an attached cyt c subunit. The cyt binds to the reaction center through an electrostatic interaction between a positively charged patch arround the heme crevice of cyt c2, and a negatively charged patch on the periplasmic face of the reaction center. The complementarity of these two faces can be nicely seen in the following two Figs.
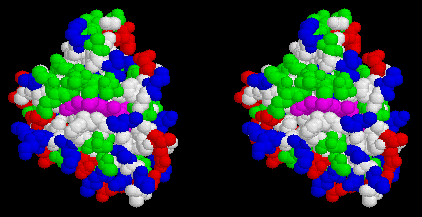
Stereo view of the surface of cytochrome c2 from Rb. sphaeroides, looking down on the heme cleft. Heme is magenta, hydrophobic residues are white, polar residues are green, acidic residues are red, basic residues are blue. Note the ring of basic residues forming the positively charged docking domain.
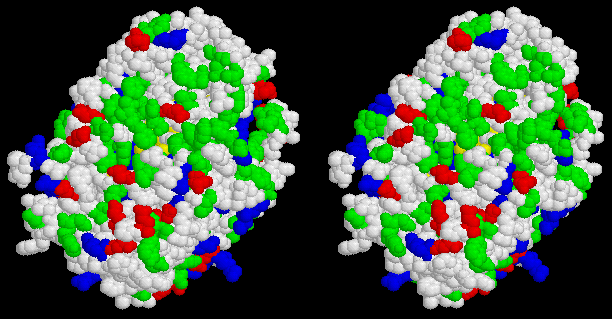
Stereo view of the surface of the reaction center from Rb. sphaeroides looking down from the periplasm. BChl is yellow, hydrophobic residues are white, polar residues are green, acidic residues are red, basic residues are blue. Note the ring of acidic residues forming the negatively charged docking domain.
As a consequence of this electrostatic binding, the reaction of cyt c2 with the RC has the following characteristics:
- The cyt c2 binds with an high affinity (KD ~1µM at low ionic strength).
- At low ionic strength, and appropriate [cyt c2], cyt oxidation following flash activation is first-order (reflecting the bound substrate). The reaction in vivo is also first-order, suggesting that at the local concentration and ionic strength, cyt c2 is bound.
- At high ionic strength, and/or low [cyt c2], the reaction is second-order, reflecting the reaction of the cyt from the unbound state.
- The second-order rate constant at low ionic strength is ~3 x 108 M-1 s-1, but this value is strongly dependent on ionic strength.
The cytochrome c subunit of the Rps. viridis reaction center
The cytocrome c subunit contains 4 hemes, two of high potential (H, ~300 mV)
and two of low potential (L, ~0 mV). The hemes are arranged in a H-L-H-L configuration, with the highest potential heme closest to the (BChl)2 (see previous lecture for a picture). Electron transfer from the nearest heme has t½ ~100 ns; when both high potential hemes are reduced before flash excitation, the hole ends up at the further heme. Electron transfer may involve the intermediate low potential heme as a weakly populated intermediate.
Plastocyanin as donor to photosystem I
Plastocyanin (PC) is the donor to the special pair of photosystem I, P700. The reaction mechanism is very similar to that of cyt c2 with the bacterial RC, except that the interaction between PC and PS I likely does not involve the same polarity of electrostatic surfaces.
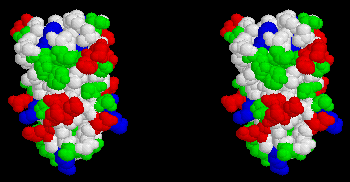
Stereo view of the surface of plastocyanin. Cu atom is hidden near top of molecule. Hydrophobic residues are white, polar residues are green, acidic residues are red, basic residues are blue. Note the two patches of acidic residues thought to form a negatively charged docking surface for interaction with cyt f or PS I.
The donor side of photosystem II - overview
Photosystem II, and its associated secondary donor complex, oxidizes water to provide the O2 which allows an aerobic biosphere to continue. The mechanism of O2-evolution remains one of the great challenges of biological research. Much progress has been made over the last decade, but much remains to be discovered.
Research on photosystem II and the mechanism of O2-evolution spans a wide range of techniques and disciplines. Much recent work has concentrated on application of various spectroscopies to an exploration of the function and structure of the Mn-complex which is central to the water oxidation process. That work is covered briefly in Lecture 24. In this brief overview, we will consider biochemical and structural aspects.
Click here to review the structure through a Chime tour of a model of photosystem II.
The model structure contains only the D1 and D2 subunits of photosystem II, for which the L and M subunits of the bacterial reactions centers provides a template. The protein in the membrane is a more complex structure, with an antenna of chlorophyll binding proteins (CP43, CP47), additional chlorphyll proteins which interface with the bulk antenna of LHCII (CP24, CP26, CP29), two subunits of cytochrome b-559 (which may serve a role in photoprotection), three extrinsic proteins, the OEEP complex (Oxygen Evolution Enhancing Proteins,- 17, 22 and 33 Kd extrinsic subunits, shown as O, P, Q below), act as ionic concentrators to maintain a suitable ionic environment at the Mn-cluster, and a number of additional subunits of uncertain function.
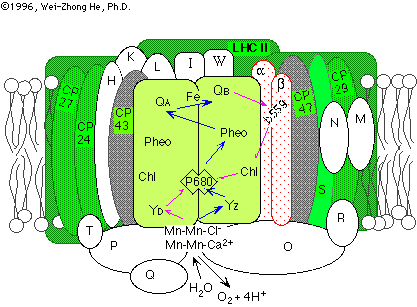
(image taken from a nice review of the photosystems by Wei-Zhong He & Richard Malkin.
With the development of a relatively pure preparation of photosystem II (the BBY prep of Berthold, Babcock and Yoccum), which retained full activity for oxygen evolution, great progress has been made in understanding the biochemistry. The smallest complex which retains photochemical function consists of D1, D2, cyt b-559, and possible other small subunits. The smallest complex which retains a normal O2-evolution contains in addition, CP43, CP47 (the core antenna), and the OEEP complex. The latter can be removed by various salt-washing treatments, and O2-evolution at normal salt concentrations is lost, but can be largely restored by addition of Ca2+ and Cl- at high concentrations.
Recently, progress has been reported on the crystallographic determination of structures for PS II. Kühlbrandt and colleagues, in collaboration with Barber's group, have reported a preliminary structure at about 8 Å resolution using electron diffraction of 2-D crystals. In combination with electron micrscopy of negatively stained images, and image averaging, they have generated a low resolution structure, which is summarized in the picture below:
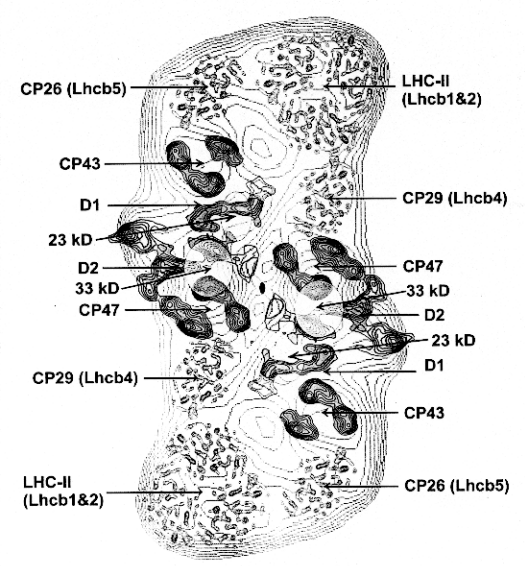
From Barber J. (1998) Photosystem 2. Bioch. Biophys Acta 1365, 269-277.
A second effort is underway in Witt's group in Berlin, where preliminary reports of 3-D crystals, which diffract to better than 6 Å were discussed at the International Congress on Photosynthesis in Budapest (1998).
YZ, the secondary donor to P+680
Work from the late 1960s identified two free radical signals in chlorolasts by room temperature EPR spectroscopy. Signal I was identified as P+700, the photosystem I primary donor. Signal II was a broader signal with side "lobes", centered around g=2.001, shown to be associated with photosystem II. Much subsequent work has shown that Signal II has two components, identified from the decay as Signal IIs (slow) and Signal IIf (fast). The latter was observed only in preparations with an inhibited O2-evolution, leading to the concept that it represented an intermediate between the OEC and P+680. With the development of faster time resolution in EPR, a Signal IIvf (very fast) was detected in O2-evolving preparations, and the decay was time-resolved to allow the first measurements of kinetics in the donor side electron transfer chain.
With the availability of sequences, and the application of molecular engineering to photosystem II, it was demonstrated that Signal IIs came from a redox active tyrosine-160 in D2 (YD), and Signal IIf (or IIvf) from tyrosine-161 in D1 (YZ). Time resolution in the ns range has now been achieved using near UV kinetic spectrophotometry, allowing resolution of the kinetics of oxidation and reduction of YZ, and a demonstration that the oxidation kinetics match the reduction of P+680, and the reduction kinetics the oxidation of the S-states. The position of these residues in relation to the Mn-cluster and P680 can be seen in the structural model of photosystem II. Further discussion of the spectroscopy of YZ can be found in Lecture 25.
Spectroscopy of Yz
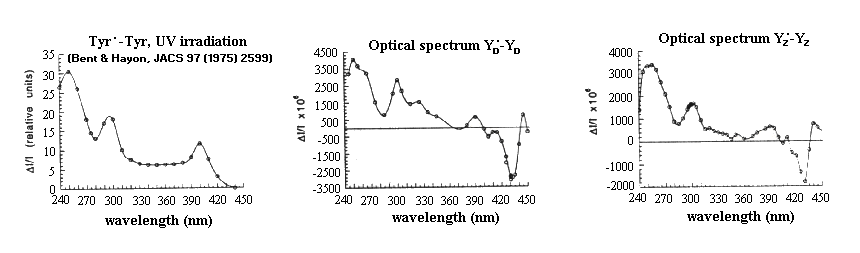
Difference spectra (Tyr· - Tyr) in the near UV attributable to:
- Left: the free radical produced by UV irradiation of tyrosine, and
- the tyrosine free-radicals of photosystem II
- Center: Tyr-160 of D2, YD,
- Right: Tyr-161 of D1, YZ
Note that the "derivative" band between 420 and 450 nm is due to a local electrochromic effect attributed to the charge on YZ+.
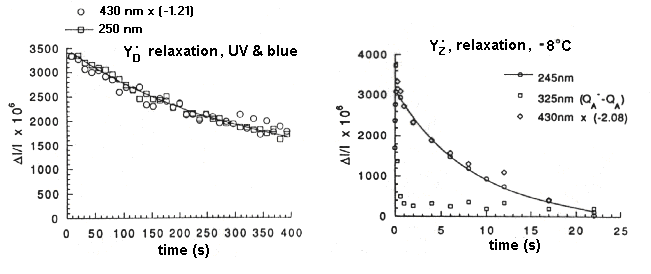
The kinetics of relaxation of the free-radical species after flash illumination.
- Left: Relaxation of YD+. Traces measured at the radical band (250 nm), and at the peak of the electrochromic band (430 nm), show the same kinetics.
- Right: Relaxation of YZ+. Traces measured at the radical band (245 nm), and at the peak of the electrochromic band (430 nm), show the same kinetics; the trace at 325 nm is due to the QA-, and the kinetics show the electron transfer to QB. The backreaction measured here is that from QB- to YZ+. (Note that the temperature is -8° C)
The EPR spectrum of YZ+.
The EPR spectrum of YZ+ is normally measured as the light-dark difference spectrum after a relatively short dark time, so that the spectrum due to YD+, which is relatively stable in the dark (see above, left), can be subtracted out. In these experiments from Babcock's lab, a mutant strain lacking YD was used, so that the spectrum due to YZ+ could be seen without overlap. This made it possible to compare spectra from preparations grown in the presence of deuterium labelled tyrosine, so as to obtain information about the orientation of the ring substituents.
Reference
Diner, B., Tang, X.-S., Zheng, M., Dismukes, C., Force, D.A., Randall, D.W. and Britt, R.D. (1995) Environment and function of the redox active tyrosines of photosystem II. In "Photosynthesis: from Light to Biosphere", ed. by Mathis, P., Vol II, pp. 229-234. Kluwer Academic Publ. Dordrecht.
The Mn-complex
Treatments which disrupt the donor side under mildly reducing conditions lead to loss of the tightly bound Mn of the OEC (Oxygen Evolving Complex). By quantifying the loss of Mn with the loss of activity on treatment with hydroxylamine, it has been shown that 4 Mn per photosytem II are essential for O2-evolution. If the Mn is removed with careful treatment, the complex can be restored by incubation with MnCl2 under appropriate conditions, Restoration requires light, which allows P+ to oxidize a Mn2+ bound at a high affinity site on D1. This site is lost if aspartate-170 is changed to a non-liganding residue.
More about the spectroscopy of the Mn-complex can be found under Lecture 25.
Much information about the involvement of specific residues in binding Mn and/or Ca2+ at the active site has come from molecular engineering by mutagenesis. In D1, residue changes giving altered O2-evolution or Mn-binding in loop ab (D59, D61, E65), loop cd (D170, E189, H190), and the C-terminal span (H332, E333, H337, D342, and the terminal -COO- of A344) identify potential ligands. Residue Y161 provides the secondary donor (Z), D170 is necessary for binding of Mn with high affinity in the assembly of the active OEC, but probably does not provide a ligand to the Mn-cluster, H198 is a possible ligand to the putative [Chl]2, and H190 is essential for O2-evolution, and a potential Mn-ligand. H195 as non-essential. These residues are in spans where the structure is unfortunately ill-defined by homology with the bacterial reaction centers. In D2, only E70 in loop ab looks like a good candidate.
See:
Debus, R. J. (1992) The manganese and calcium ions of photosynthetic oxygen evolution. Biochim Biophys Acta 1102, 269-352
for a comprehensive review
The intermediate electron transfer chains
The bc1-complex
An extensive set of links to the ubiquinol:cyt c oxidoreductase, Cytochrome bc1-complex,- Complex III can be found here. These include experimental work on the complex from photosynthetic bacteria, information about the X-ray crystallographic structure of the mitochondrial complexes as this becomes available, and a working model of the Q-cycle.
The b6f-complex
General
The complex has the same complement of redox centers as the bc1-complex, but the cytochrome b heme(s) for historical reasons are called cytochrome b6, and the heme c center is called cytochrome f. The redox centers are bound in three subunits which show homology with the three redox subunits of the bc1-complex. The protein corresponding to cytochrome b is separated into 2 subunits, with a "split" in the de loop.
- Cytochrome b, which shows strong homology with the N-terminal section of bacterial or mitochondrial cyt b, and shows a predicted folding pattern corresponding to the span from the N-terminus to the de span between transmembrane helices D and E. This segement contains both of the helices with putative histine ligands for the two hemes. Helix D has an extra residue inserted between the liganding histidines.
- The second part is called subunit IV (SUIV), and corresponds to the C-terminal part of bacterial cyt b, from the "split" in the de loop to a C-terminus truncated before the H-helix.
Structure
The structure of a soluble cytochrome f (which has lost the membrane anchor provided by the C-terminal helical span) has been solved at high resolution by Martinez, S.E., Smith, J.L., Huang, D., Szczepaniak, A. and Cramer, W.A.
Click here for a brief tour of the structure of cytochrome f, using Chime.
Mechanism
The mechanism of the b6f-complex is still controversial. The experimental data can be interpreted as showing that the complex operates through a modified Q-cycle, just like the that for bc1-complex, but with adjustments in rate constants and equilibrium constants as follows (Kramer and Crofts mechanism):
- The Em values for cytochromes bH and bL are lower, with values of approx. -50 mV and -150 mV respectively.
- The semiquinone at the Qi-site is much less stable (Em < -50 mV for Q/SQ couple), so that reduction by cyt bH does not occur significantly, and the site operates effectively by transferring two electrons only when both b-cytochromes are reduced.
- The complex turns over from the fully reduced state by an "autocatalytic" mechanism.
Other groups (Joliot, Cramer, Fernandez-Velasco) have suggested that a semiquinone cycle operates. In this model, oxidation of QH2 at the Qo-site generates a semiquinone (as in the Q-cycle), but if the low potential b-cytochrome is reduced, the semiquinone leaves the site as an anion, and is oxidized at the Qi-site to give an electrogenic reaction involving transfer of the semiquinone anion across the membrane. This mechanism has been proposed as an alternative to the autocatalytic mechanism to explain how the complex turns over from the fully reduced state .
See:
Cramer, W.A., Soriano, G.M., Ponomarev, M., Huang, D., Zhang, H.,Martinez, S.E. and Smith, J.L. (1996) Some new structural aspects and old controversies concerning the cytochrome b6 complex of oxygenic photosynthesis. Ann. Rev. Plant Physiol. Plant Mol. Biol. 47, 477-508.
for a recent review.
Ferredoxin and flavodoxin as mediators of electron flow from photosytem I to reductive metabolic pools
CO2 is fixed by the enzyme ribulose-bis-phosphate carboxylase-oxygenase (RUBISCO). The product of the carboxylation process is 3-phosphoglycerate (3-PGA), which is phosophorylated by ATP and reduced to glyceraldehyde-3-phosphate by NADPH.
ribulose-bisphosphate + CO2 --> 2 3-PGA
2 x ( 3-PGA + ATP --> 1,3-diphosphoglycerate
1,3-diphosphoglycerate + NADPH --> glyceraldehyde-3-phosphate + NADP+ + phosphate + H+ )
Electrons from photosystem I are delivered to NADPH, via a soluble redox protein,- either ferredoxin (in higher plants, and in algae under Fe-sufficient conditions), or flavodoxin (in algae under Fe-depleted conditions), and ferredoxin:NADP reductase (FNR).
(PSI FESA/B)- + Fd ---> (PSI FESA/B) + Fd-
2Fd- + NADP+ + H+ --(FNR)--> 2Fd + NADPH
©Copyright 1996,
Antony Crofts, University of Illinois at Urbana-Champaign,
a-crofts@uiuc.edu